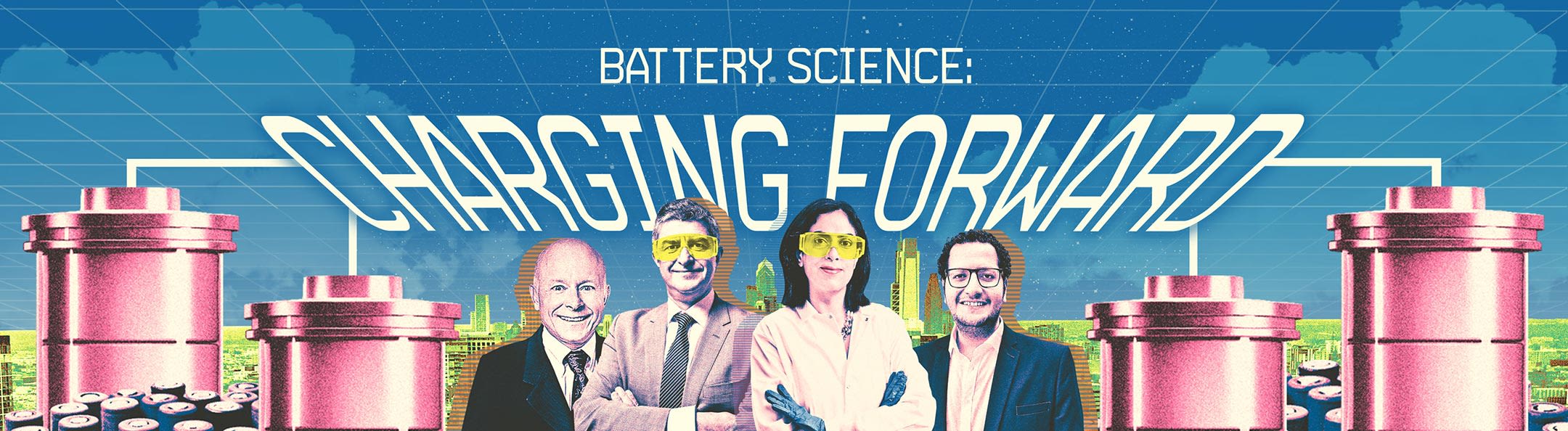
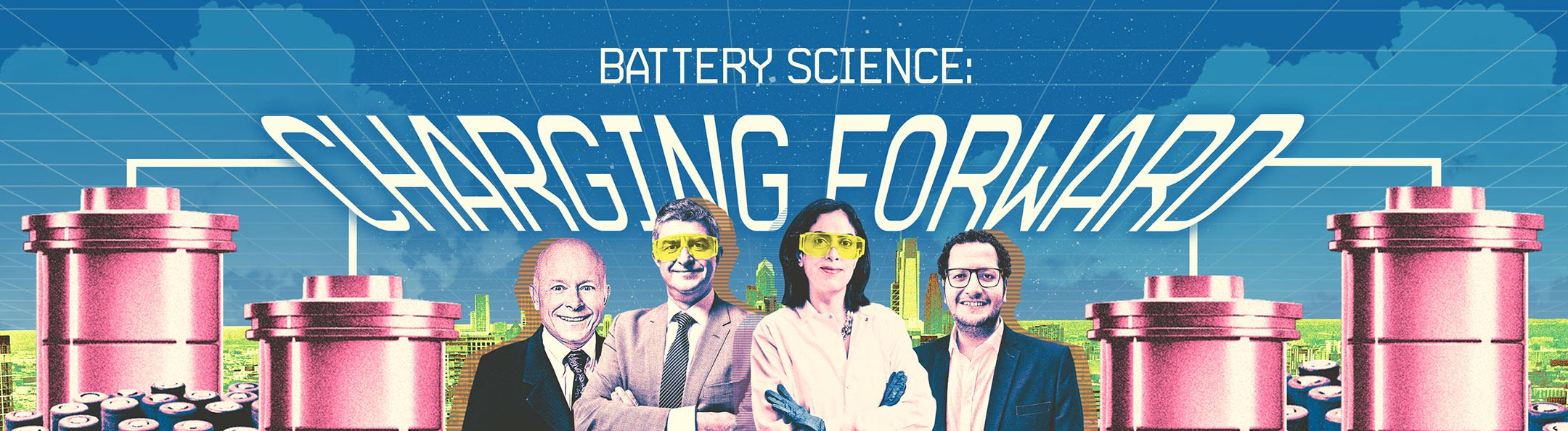
Content sponsored and provided by EXEL, Drexel University’s Research Magazine.
Content sponsored and provided by EXEL, Drexel University’s Research Magazine.
Battery Science: Charging Forward
Discoveries by Drexel engineers and materials scientists are creating opportunities for better electrical vehicles, textile electronics and safe hydrogen energy.
Breakthroughs in battery science by Drexel University engineers are paving the way for new tech that can solve big energy problems. In this package of stories from Drexel’s College of Engineering, hear about the worldwide hunt for a killer app for MXenes…learn about a new method of making nanomaterials that could be a source safe hydrogen energy…follow one researcher’s path toward commercializing a drone battery…and discover how researchers are keeping electric vehicle batteries charged and cooled.
THE PATH TO LIFT-OFF
In the mid-1980s, Sony executives were under pressure.
The corporation was racing to develop a high-density, rechargeable battery that could power its electronics again and again. But Union Carbide had just pulled out of their R&D joint venture and a rival Japanese firm already had a battery prototype, recounts Charles J. Murray in the book “Long Hard Road: The Lithium-Ion Battery and the Electric Car.” In 1987, the head of Sony’s battery development subsidiary, Keizaburo Tozawa, shifted to high gear, assigning six separate research groups to focus solely on this mission. Two and a half years later, Sony announced its “dream battery,” a rechargeable lithium-ion battery that was safer and more powerful than its competitors.
Fast-forward three decades: These revolutionary lithium-ion batteries, first used in Sony camcorders, now power everything from the smartphones in our pockets to the electric cars in our driveways. They have seen vast improvements over the past 30 years — most notably in the amount of energy they can store — and are still considered the gold standard rechargeable battery.
But every technology has its limits, and the lithium-ion battery is bumping up against its limits in the electric vehicle market. For a lithium-ion battery to store more energy — to run longer between charges — it must weigh more. While that increased weight isn’t much of a problem in small electronics, it is an issue in vehicles. The lithium-ion battery powering a Tesla, for instance, weighs more than 1,000 pounds and accounts for as much as half of the car’s total cost.
“That’s not sustainable,” says Vibha Kalra, who is the George B. Francis Chair Professor and director of the PhD Program in Drexel’s Department of Chemical and Biological Engineering within the College of Engineering. “When you try to translate the same technology into larger vehicles, it’s not going to be sustainable with respect to both weight and cost. A pickup truck would require at least twice the battery storage of a sedan. A commercial truck could require almost tenfold.”
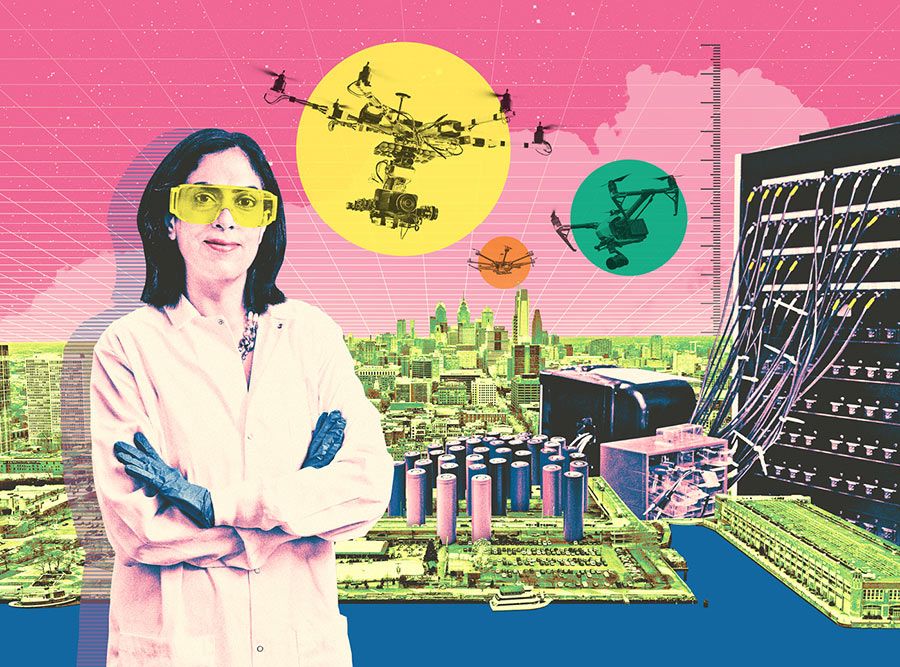
Kalra is working to bridge this gap. In her decade-long journey to create and commercialize a new generation of rechargeable batteries, Kalra has performed hundreds of experiments, interviewed dozens of industry experts, received close to $7 million in grants and funding, logged countless hours working with tech commercialization experts in Drexel’s Office of Research & Innovation, and secured three patents, with 11 more pending.
Though the work isn’t over, the path of Kalra’s research discovery from lab bench to a top commercial prospect exemplifies the power of academic entrepreneurship in supercharging faculty careers and expanding the University’s research enterprise.
“It gives a good picture of what it’s like to take a really early-stage, interesting technology to the point where it’s ready to make its way into a company,” says Robert McGrath, senior associate vice provost for intellectual property and agreements in Drexel Applied Innovation, the technology transfer division of the Office of Research & Innovation. “Getting to market [is hopefully] not far behind.”
THE DISCOVERY
Chemical engineers have long been intrigued by the potential of combining lithium with sulfur to make a new kind of battery. The opportunity is enticing: Lithium-sulfur batteries have the potential to store more than five times the energy of their lithium-ion counterparts. As a widely abundant material, sulfur is super cheap. It’s also benign, unlike some heavy metals and materials that face environmental and humanitarian issues as well as looming shortages.
All of this promises an affordable, abundant, lighter, greener battery — with spectacular performance in everyday electronics. Imagine running your laptop for three times as long, or getting the same run time from a laptop that weighed a third less. An electric car with a lithium-sulfur battery would have a range of 1,500 miles, versus 500 miles for a lithium-ion battery of the same weight.
But Kalra and other researchers were stumped by a critical obstacle to using sulfur. Both lithium-ion and lithium-sulfur batteries contain a liquid, called electrolyte, that transports lithium ions from one side of the battery to the other, enabling its charge and discharge cycle. Most experimenters studying lithium-sulfur batteries have favored ether electrolyte because it plays well with sulfur. However, it has a low boiling point, making it too dangerous for practical use.
Kalra and her PhD student decided to ditch ether electrolyte in favor of carbonate electrolyte, which has been used in lithium-ion batteries for 30 years.
“It’s the path of least resistance,” Kalra explains. “Rather than pushing for the industry adoption of a new electrolyte, our goal was to make a cathode that could work in the pre-existing Li-ion electrolyte system.”
Of course, it isn’t as easy as swapping one electrolyte for the other. When Kalra first put carbonate electrolyte in an experimental lithium-sulfur battery, the battery shut down after its first cycle. To counteract the adverse reaction between sulfur and carbonate electrolyte, the team tried to isolate sulfur from the carbonate by enmeshing it in a carbon nanofiber substrate using a vapor deposition technique.
They didn’t get the result they expected. But as they explain in their paper published in Communications Chemistry, what they got instead was a game changer: a rare form of crystalized sulfur, called monoclinic gamma sulfur, that is stable at room temperature. Long-term stability of this form of sulfur had never been achieved in a lab before.
“As we began the test [of the cathode], it started running beautifully — something we did not expect,” Kalra recalls. “In fact, we tested it over and over again — 50–100 times — to ensure we were really seeing what we thought we were seeing.”
When the team put their stable sulfur into a battery, they were able to cycle it 4,000 times, or the equivalent of 10 years of use, without losing performance. In contrast, a typical lithium-ion battery can cycle 2,000 times and has only a third of the energy density.
With her discovery confirmed, Kalra called Drexel Applied Innovation…
Read the rest of this story in Drexel University’s EXEL Magazine at Battery Science: Charging Forward. While there, be sure to also check out the included articles The Great Conductor; A Simpler, Greener Wonder Material?; Charging While Driving; and Behold the Battery Chassis to learn more about how Drexel engineers and materials scientists are working to reshape energy technology.
This content is sponsored by EXEL, Drexel University’s Research Magazine and developed by Inside Higher Ed's sponsored content team. The editorial staff of Inside Higher Ed had no role in its creation.